A Quantum Leap in Efficiency: 3 Orders of Magnitude Improvement in On-Chip Supercontinuum Generation
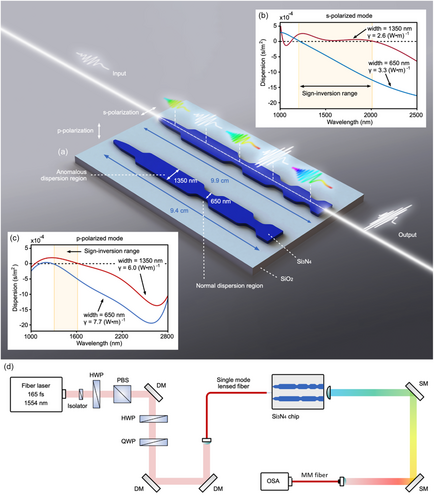
Researchers have achieved a groundbreaking advancement in the field of ultraefficient on-chip supercontinuum generation, paving the way for the development of portable and mechanically stable medical imaging devices, chemical sensors, and light detection and ranging (LiDAR) systems. By employing sign-alternating dispersion in a CMOS-compatible silicon nitride waveguide, the scientists have drastically lowered the input energy requirements for integrated supercontinuum generation, unlocking the potential of this cutting-edge technology and opening new doors for a wide range of applications.
The Challenge of Current Supercontinuum Generation Schemes
Supercontinuum generation is a process that broadens the range of light wavelengths, allowing for a wide variety of applications such as medical imaging devices, chemical sensors, and LiDAR systems. However, existing methods for generating supercontinuum light on small chips face efficiency challenges, which hinder their integration into compact and portable devices.
The efficiency of a supercontinuum generation system is crucial in determining how effectively it can be integrated into a compact device. Unfortunately, most current techniques require a significant amount of energy input to function effectively, making it difficult to produce supercontinuum light sources that can be incorporated into small-scale, portable systems. As a result, researchers have been searching for ways to improve the efficiency of these systems, ultimately aiming to develop fully integrated on-chip supercontinuum sources.
Enabling On-Chip Integrated Supercontinuum Laser Sources
By lowering the pulse energy requirement for large-bandwidth on-chip supercontinuum generation at high spectral power, researchers have enabled the use of chip-integrated laser sources, such as mode-locked heterogeneously or hybrid-integrated diode lasers, as a pump source. This development paves the way for fully integrated on-chip high-bandwidth supercontinuum sources, opening new doors for applications like frequency combs, hyperspectral LiDAR, optical coherence tomography (OCT), and nonlinear optical pulse compression.
Design and Experimental Demonstration
In simpler terms, researchers have developed and tested a new type of waveguide that significantly improves the generation of a wide range of light wavelengths. By using alternating dispersion in silicon nitride waveguides, they achieved a dramatic enhancement in the efficiency of generating a broad spectrum of light. This enhancement is up to 2800 times more efficient compared to current methods. As a result, the energy needed to achieve this effect has been greatly reduced, making it more feasible to integrate into portable devices and various applications.
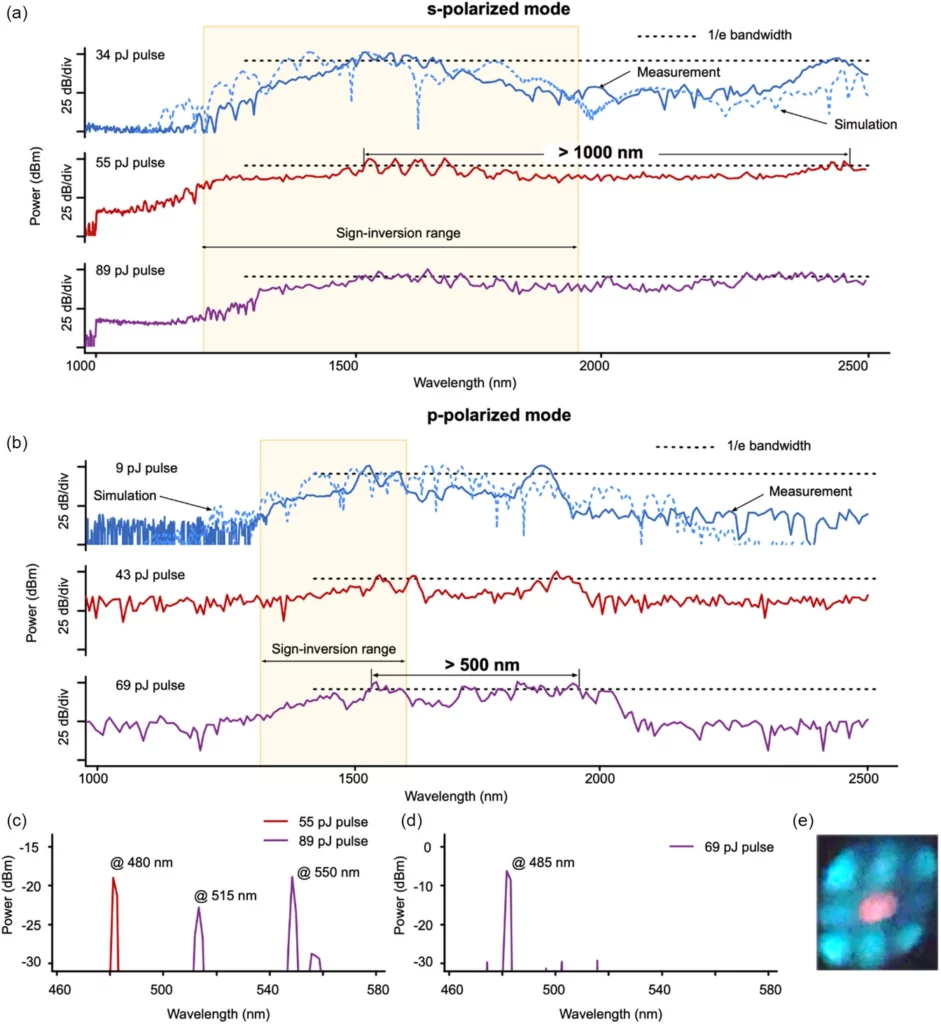
Versatility and Future Applications
Alternating dispersion waveguides can be implemented in a variety of material platforms, particularly those with high-contrast refractive index materials. As a result, researchers plan to extend the material platforms of this approach and optimize the output temporal profiles of the pulses for pulse compression applications.
Closing the Gap with Integrated Ultrafast Diode Lasers
The energy needed for the cutting-edge on-chip supercontinuum generation process is now much closer to what the latest integrated ultrafast diode lasers can provide. These diode lasers, which emit light at a specific wavelength, are a critical component in this new technology. By getting closer to the energy levels offered by these lasers, researchers can potentially develop devices that are not only more efficient but also more compatible with existing technology. This breakthrough paves the way for the creation of advanced solid-state lasers that are better suited for integration with other components, ultimately leading to more powerful and versatile devices in medical imaging, chemical sensing, and LiDAR systems.
Conclusion and Potential Impact
This groundbreaking research has the potential to revolutionize the fields of medical imaging, chemical sensing, and LiDAR, leading to the development of portable and mechanically stable devices with a wide range of applications.