Beam Splitters: Types, Applications, and Selection
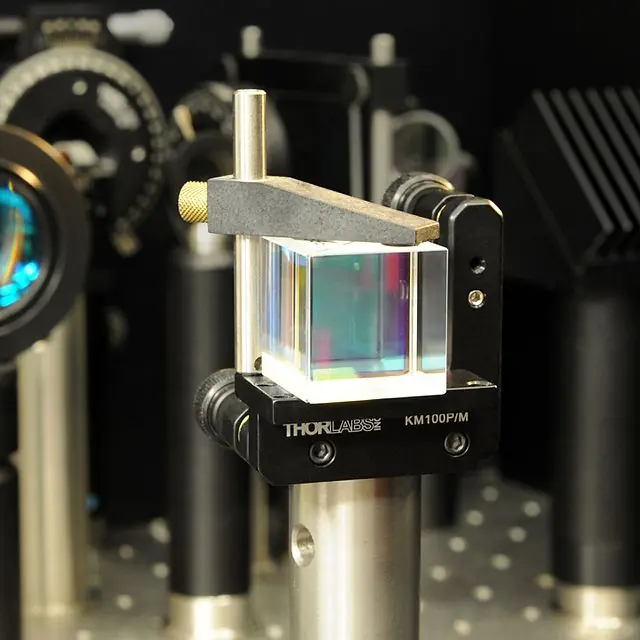
Beam splitters are an essential component in modern optics. They play a critical role in many fields, including scientific research, medical imaging, entertainment, and telecommunications. In this article, we will explore the various types of beam splitters, how they work, their applications, and advancements in beam splitting technology.
Types of Beam Splitters
There are several types of beam splitters, each designed for specific applications. The most common types of beam splitters are polarizing, non-polarizing, dichroic, cube, and plate beam splitters.
Polarizing beam splitters only reflect light with a specific polarization while allowing the remaining light to pass through. They are used in applications that require separating light based on polarization, such as polarizing microscopes and laser optics.
Non-polarizing beam splitters are designed to split light evenly regardless of polarization. They are used in applications that require even distribution of light, such as laser beam alignment, and beam sampling.
Dichroic beam splitters work by reflecting light of certain wavelengths while transmitting others. They are commonly used in fluorescence microscopy, where the beam splitter is used to separate excitation light from emitted fluorescence.
Cube beam splitters are made from two prisms cemented together at a specific angle. They work by reflecting part of the incident light and transmitting the rest. They are used in interferometers, polarimeters, and other applications that require splitting the light at a specific angle.
Plate beam splitters are made from a single piece of glass or other transparent material with a thin layer of metal deposited on one side. They work by reflecting part of the light and transmitting the rest. They are commonly used in applications that require beam splitting with high reflectivity.
How Beam Splitters Work
The function of beam splitters is to divide an incoming beam of light into two or more separate beams. The splitting can be achieved by reflecting or transmitting part of the incident light, and the amount of light that is reflected or transmitted depends on several factors, including the angle of incidence, polarization, and the type of beam splitter used.
When a beam of light hits a beam splitter, some of the light is reflected, while the remaining light is transmitted. The reflected light follows the law of reflection, while the transmitted light follows the law of refraction. Depending on the design of the beam splitter, the splitting of the beam can be adjusted to produce a specific ratio of reflected and transmitted light.
The angle of incidence and the polarization of the incoming beam also affect how beam splitters work. The amount of light that is reflected or transmitted changes with variations in the angle of incidence, and the polarization of the incoming beam can be used to control the amount of light that is reflected or transmitted. Polarizing beam splitters, for example, are designed to split light based on polarization, and they only reflect light with a specific polarization while allowing the rest of the light to pass through.
Applications of Beam Splitters
Beam splitters have numerous applications in various fields. In microscopy, beam splitters are used to separate excitation and emission light in fluorescence microscopy. They are also used in confocal microscopy to split the light between the specimen and the detector.
In interferometry, beam splitters are used to divide a single beam of light into two, which are then combined to create an interference pattern. This technique is used in optical testing, vibration analysis, and other applications that require precise measurement of distances.
In telecommunications, beam splitters are used to combine or split optical signals. They are used in fiber optic networks, where they help in routing and distributing signals.
In the entertainment industry, beam splitters are used in projectors and other lighting equipment to split light into multiple beams, creating stunning visual effects.
Choosing the Right Beam Splitter
Choosing the right beam splitter is crucial for achieving optimal performance and accuracy in various applications, including scientific research, medical imaging, and telecommunications. Several factors should be considered when selecting a beam splitter, including wavelength range, polarization, angle of incidence, and power handling.
One of the most important factors to consider is the wavelength range. It is essential to choose a beam splitter that can handle the wavelength range of the light source used in the application. Using an incompatible wavelength range can lead to significant losses in transmission and reflection, affecting the accuracy and reliability of the results.
Polarization is another critical factor to consider. Some applications require specific polarization, while others require even distribution of light regardless of polarization. It is essential to select the appropriate polarizing or non-polarizing beam splitter that fits the application’s needs.
The angle of incidence also plays a crucial role in beam splitter selection. The angle of incidence can affect the amount of light that is reflected or transmitted by the beam splitter. Therefore, it is essential to choose a beam splitter that is optimized for the specific angle of incidence used in the application.
Finally, power handling is another important consideration. Beam splitters can experience thermal effects when exposed to high power levels, leading to damage or degradation of performance. It is essential to choose a beam splitter that can handle the power levels of the application without affecting performance.
Avoiding common mistakes when selecting a beam splitter, such as using an incompatible wavelength range or neglecting polarization and angle of incidence requirements, is crucial to achieving optimal performance and accurate results. Taking into account these factors and ensuring compatibility with other components in the system can help select the right beam splitter for the application’s needs.
Advancements in Beam Splitting Technology
The continuous advancements in beam splitting technology have revolutionized the field of optics. One of the most recent breakthroughs is the use of metasurfaces, which are ultra-thin structures that can manipulate light at the nanoscale level. Metasurfaces provide exceptional control over the amplitude, phase, and polarization of light, making them ideal for beam splitting applications.
Metasurface-based beam splitters are highly efficient, compact, and can operate over a wide range of wavelengths. They have the potential to replace traditional beam splitters, which are bulkier and less efficient. Researchers are also exploring the use of metasurface-based beam splitters in applications such as holography and optical communications.
Future advancements in beam splitting technology are likely to focus on improving efficiency, reducing size, and enhancing the range of wavelengths that can be handled by beam splitters. The development of metasurface-based beam splitters is a significant step towards achieving these goals.
Conclusion
Beam splitters are a fundamental building block in modern optics, and their importance cannot be overstated. They have numerous applications in various fields, from scientific research to entertainment. Selecting the right beam splitter is crucial for achieving the desired results, and advancements in beam splitting technology are continuously improving their performance.
The use of metasurfaces has led to the development of highly efficient and compact beam splitters that can operate over a wide range of wavelengths. These new technologies have the potential to replace traditional beam splitters and lead to new applications in holography, optical communications, and other fields. Future advancements in beam splitting technology will undoubtedly bring even more exciting developments, further expanding our ability to manipulate light for various purposes.