Optical Resonators: Principles, Types, and Applications in Modern Technology
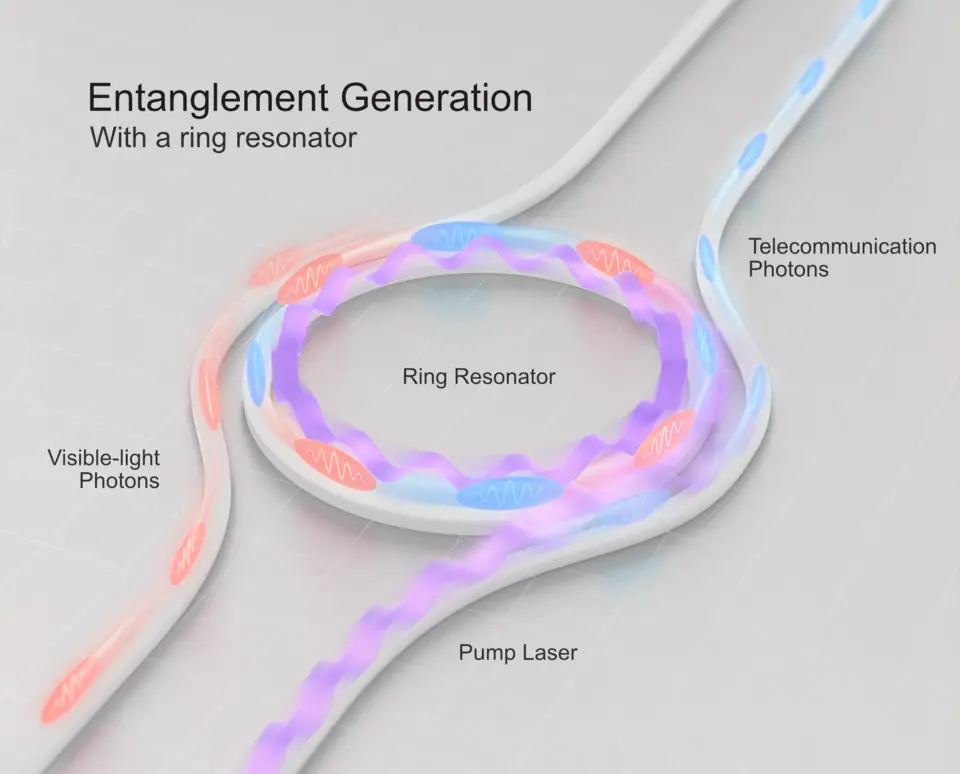
Optical resonators, sometimes referred to as optical cavities, are essential components in various modern technologies, from lasers and optical sensors to telecommunications systems. By trapping and circulating light within a defined space, an optical resonator can amplify or manipulate light waves, resulting in a wide array of practical applications. This article delves into the principles behind optical resonators, their diverse types, and their ever-growing significance in today’s technological landscape.
Principles of Optical Resonators
The fundamental principle underlying optical resonators is resonance, a phenomenon where light waves interact constructively within a confined space, leading to an amplification of the light’s intensity. To better understand this concept, let’s explore the key elements involved in the operation of optical resonators.
- Light waves and interference: Light waves can undergo constructive or destructive interference when they overlap. In constructive interference, the waves combine in phase, resulting in a larger amplitude, while in destructive interference, out-of-phase waves cancel each other out. Optical resonators are designed to maximize constructive interference, ensuring that only specific wavelengths build up inside the cavity.
- Role of mirrors in optical resonators: Mirrors form the basis of most optical resonators by reflecting light waves back and forth within a cavity. Highly reflective mirrors help minimize losses, thereby allowing light of particular wavelengths to resonate. The spacing and alignment of the mirrors determine which wavelengths can form standing waves within the resonator.
- Formation of standing waves: When light waves are confined within a resonator and undergo constructive interference, they form a stable pattern known as a standing wave. These waves have stationary nodes and antinodes, which correspond to points of minimal and maximal intensity, respectively. The formation of standing waves in a resonator is crucial for achieving resonance and amplification of specific wavelengths.
- Resonant frequencies and modes: Optical resonators support multiple resonant frequencies, also called modes. These modes correspond to the different wavelengths that can form standing waves within the cavity. The mode structure depends on the resonator’s geometry, mirror properties, and cavity length. In many applications, it is desirable to operate the resonator in a single mode to achieve precise control over the output wavelength.
- Quality factor (Q-factor): The Q-factor is a measure of an optical resonator’s ability to store energy and maintain resonance. A higher Q-factor indicates lower losses and a more efficient resonator. The Q-factor is influenced by factors such as mirror reflectivity, absorption, and scattering within the resonator. In certain applications, such as precision metrology and sensing, high-Q resonators are essential for achieving the desired performance.
- Gain and amplification: In certain optical resonators, such as those used in lasers, a gain medium is introduced to provide amplification of the light waves. The gain medium, when stimulated, emits photons that are coherent with the circulating light. This process leads to amplification of the resonant wavelengths and enables the resonator to produce a high-intensity output.
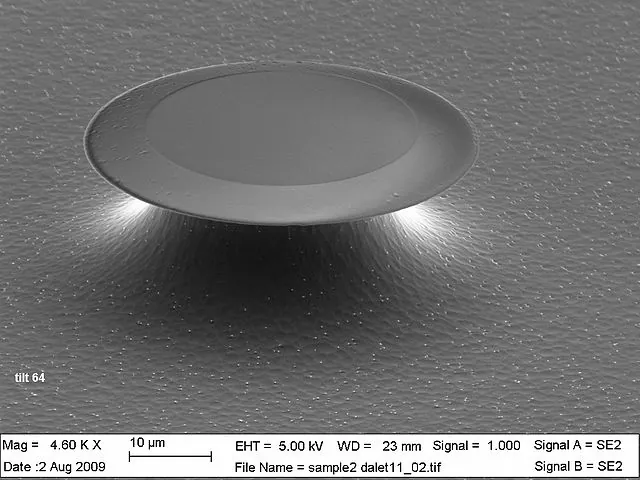
By leveraging these principles, optical resonators can manipulate and amplify light waves in a controlled manner, enabling a wide range of applications in modern technology.
Types of Optical Resonators
Optical resonators come in various forms, each with its unique characteristics and applications. Some of the most common types include Fabry-Perot, ring, spherical, and cylindrical resonators, while more advanced resonator designs like whispering gallery mode resonators and photonic crystal resonators have emerged in recent years.
- Fabry-Perot resonator: The Fabry-Perot resonator consists of two parallel mirrors, forming a cavity where light bounces back and forth. This resonator type allows for precise wavelength control due to its ability to selectively enhance specific wavelengths that satisfy resonance conditions. Fabry-Perot resonators are widely used in spectroscopy, lasers, and telecommunication systems for wavelength filtering and stabilization.
- Ring resonator: The ring resonator features a closed-loop configuration, enabling light to circulate in a circular path. The ring design allows for minimal loss due to the absence of sharp corners and the possibility of achieving higher Q-factors compared to other resonator types. Ring resonators find applications in integrated optics, filtering, and optical switching, as well as in nonlinear optics for frequency conversion and parametric processes.
- Spherical and cylindrical resonators: These resonators are characterized by their distinct curved geometries, which offer unique advantages for light confinement and the formation of whispering gallery modes. These modes result from light waves that propagate around the resonator’s inner surface with minimal loss. Spherical and cylindrical resonators are utilized in cavity quantum electrodynamics (QED) experiments, high-Q resonators for ultra-sensitive measurements, and single-photon studies. They also find use in frequency-stabilized lasers and optical biosensors.
- Whispering gallery mode (WGM) resonators: WGM resonators are a class of high-Q optical resonators that exploit the unique properties of whispering gallery modes. These resonators, typically microscale in size, can be fabricated using a variety of geometries such as spheres, toroids, or disks. Light waves travel along the resonator’s curved surface, with minimal loss due to total internal reflection. WGM resonators find applications in optoelectronic oscillators, highly sensitive sensors, and single-photon sources, as well as in nonlinear optics and quantum information processing.
- Photonic crystal resonators: These resonators are formed using periodic dielectric structures, known as photonic crystals, which manipulate light on a sub-wavelength scale. By creating localized defect states within the photonic crystal, optical modes can be confined, leading to resonant behavior. Photonic crystal resonators are employed in compact, highly efficient devices such as filters, modulators, and lasers. They are also being explored for use in quantum information processing and sensing applications due to their ability to confine light with extremely low losses.
Each type of optical resonator offers unique advantages and capabilities, making them suitable for a wide range of applications across various industries and scientific fields. Researchers continue to explore new resonator designs and materials to further expand the potential of optical resonators in emerging technologies.
Optical Resonator Components and Materials
Optical resonators rely on several critical components and materials, including mirrors, gain media, and coupling elements.
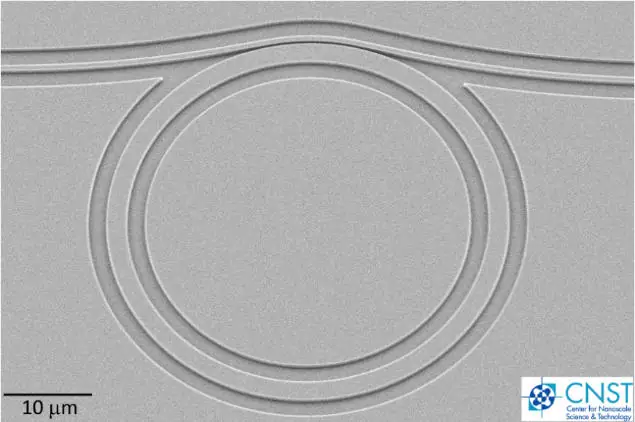
- Mirrors: Dielectric mirrors, made from alternating layers of materials with different refractive indices, offer excellent reflectivity and low absorption. Metallic mirrors, on the other hand, provide high reflectivity but may also exhibit higher absorption and dispersion.
- Gain medium: Gain media, which can be solid-state, gas-based, or liquid-based, provide the necessary amplification for the light waves circulating within the resonator.
- Coupling elements: Fiber couplers and waveguide couplers are used to introduce and extract light from the resonator cavity, ensuring efficient coupling while minimizing losses.
Optical Resonators Applications
Optical resonators have found widespread use in various industries, thanks to their versatility and ability to manipulate light. Some of the most prominent applications of these devices include lasers, optical filters, optical sensors, optical clocks and frequency combs, telecommunications, and data processing.
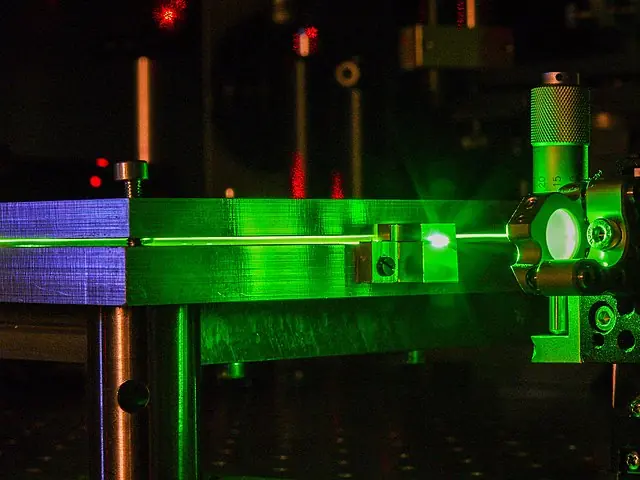
- Lasers: Optical resonators are at the core of numerous laser systems, providing essential control over wavelength, power, and beam quality. In industrial settings, lasers with optical resonators are used for cutting, welding, and engraving materials, as well as for surface treatment processes. In the medical field, they are employed for surgical procedures, dermatology treatments, and diagnostic tools like optical coherence tomography (OCT). Optical resonators are also indispensable in various scientific research settings, including laser spectroscopy, laser cooling, and the study of nonlinear optical phenomena.
- Optical filters: Resonators can function as wavelength-selective filters, critical for numerous applications. In telecommunications, optical resonators are employed in wavelength division multiplexing (WDM) systems to filter and route signals in fiber-optic networks. They also enable the creation of highly selective sensors that can detect specific wavelengths, making them suitable for monitoring environmental, biomedical, and industrial parameters. Additionally, optical resonators can act as filters in spectrometers, enhancing the accuracy and resolution of spectroscopic measurements.
- Optical sensors: Optical resonators are vital components in various types of sensors. For environmental monitoring, optical resonator-based sensors are used to track air quality, water pollution, and other factors. In biomedical applications, resonator-based sensors can detect biomolecules or monitor physiological parameters in medical diagnostics and research. In industrial process control, sensors with optical resonators can measure parameters like temperature, pressure, and chemical concentrations, ensuring process stability and safety.
- Optical clocks and frequency combs: Optical resonators play a key role in ultra-precise timekeeping and metrology. Advanced GPS technologies rely on highly stable optical resonators to maintain precise time synchronization for accurate position determination. Optical resonators are also crucial components in atomic clocks, which provide the foundation for international time standards and enable timekeeping with unprecedented accuracy.
- Telecommunications and data processing: Optical resonators have made their mark in the field of information technology. Integrated optical resonators enable high-speed communication between different parts of a microchip, reducing latency and increasing data transfer rates. Optical resonators can also be used in reconfigurable switches and routers for efficient data routing in optical networks. Furthermore, resonators have the potential to enhance data storage technologies by allowing for higher-density optical storage and faster read/write speeds.
These diverse applications showcase the importance of optical resonators in numerous industries and research fields. As technology advances, the scope and potential of optical resonators are expected to expand even further.
Emerging Technologies and Research in Optical Resonators
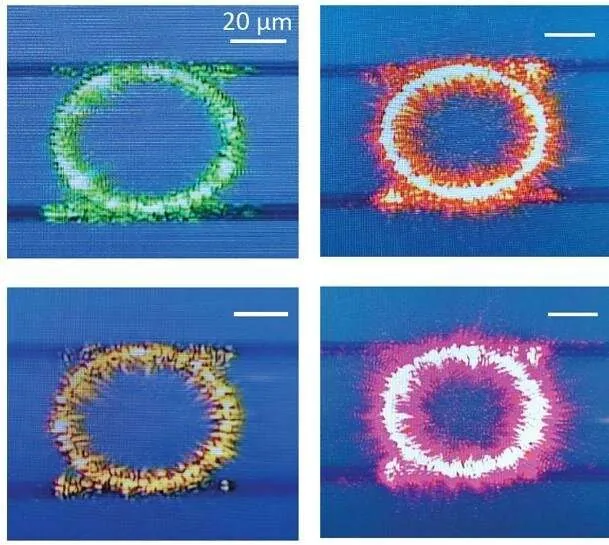
Optical resonators have experienced significant advancements in recent years, with researchers focusing on new frontiers to further unlock their potential. Some of the most promising areas of exploration include photonic crystal resonators, microresonators and on-chip integration, metamaterials, and their applications in quantum computing and communication.
- Photonic crystal resonators: These resonators utilize periodic structures with dimensions comparable to the wavelength of light to manipulate the propagation of electromagnetic waves. They have the potential to dramatically improve light confinement and control, which can result in more efficient and compact optical devices. Photonic crystal resonators can be employed in ultra-low-threshold lasers, wavelength-division multiplexing (WDM) systems, and high-sensitivity biosensors. One of the main challenges in developing photonic crystal resonators is to achieve precise control over their fabrication and the uniformity of their lattice structures.
- Microresonators and on-chip integration: With the growing demand for miniaturized and integrated photonic devices, microresonators have emerged as a promising avenue for research. These small resonators allow for high-density integration of optical components on a single chip, leading to more compact and energy-efficient systems. Microresonators have been utilized in various applications, including optical switching, signal processing, and nonlinear optics. They also hold promise for the development of lab-on-chip devices, where multiple optical functions can be performed on a single platform. Ensuring reliable and efficient coupling between microresonators and other on-chip components, as well as mitigating propagation losses, remain significant challenges for researchers.
- Metamaterials in optical resonators: Metamaterials are artificially engineered structures with unique properties not typically found in natural materials. By incorporating metamaterials into optical resonators, researchers hope to achieve unprecedented control over light-matter interactions. Metamaterial-based resonators have potential applications in super-resolution imaging, cloaking devices, and enhanced nonlinear optical processes. The fabrication of large-scale, high-quality metamaterials is a major challenge, as is the potential for increased energy losses due to absorption and scattering.
- Quantum computing and quantum communication: Optical resonators are playing a crucial role in the development of quantum technologies by facilitating precise control of single photons and enabling strong coupling between light and matter. Optical resonators have been employed in quantum computing for qubit storage and manipulation, as well as in quantum communication systems for secure data transmission. Building large-scale quantum systems with high levels of precision and stability is a complex task, requiring advances in resonator design, materials, and fabrication techniques.
Conclusion
Optical resonators have come a long way since their inception, with numerous types and applications now available across various industries. As research progresses, emerging technologies like photonic crystal resonators, microresonators, and quantum applications continue to push the boundaries of what is possible with optical resonators. The future holds exciting prospects for these versatile devices, as they continue to shape the landscape of modern technology and pave the way for new breakthroughs.