Pulsed Dye Lasers: A Deep Dive into Their Principles, Advantages, Limitations, and Uses
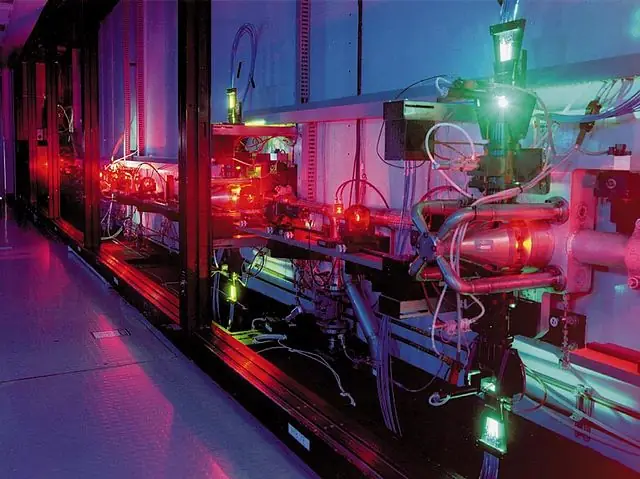
Lasers have become an essential tool in modern science and technology, with different types catering to a wide range of applications. Among them, the pulsed dye laser (PDL) stands out due to its unique properties and versatility. In this article, we will explore the principles behind pulsed dye lasers, their advantages and limitations, as well as their diverse applications in various fields.
What is a Pulsed Dye Laser?
A pulsed dye laser, often abbreviated as PDL, represents a unique class of lasers that utilize organic dye molecules dissolved in a liquid solvent as the lasing medium. To better comprehend the workings of a PDL, let’s delve into its primary components: the dye medium, the pumping source, and the resonator.
The dye medium, which imparts the tunable wavelength range to the laser, is central to the PDL’s adaptability. The pumping source, typically a flashlamp or another laser, supplies the energy necessary to excite the dye molecules. Finally, the resonator, composed of two mirrors, amplifies and directs the laser light generated within the system.
PDLs operate in a pulsed manner, using techniques like Q-switching or mode-locking to produce short, high-intensity light pulses. These methods facilitate impressive peak power output and offer precise control over the emitted laser light, making PDLs highly versatile for a wide range of applications.
Advantages of Pulsed Dye Lasers
Pulsed dye lasers offer several benefits that make them attractive for various applications. These advantages include:
- Tunable wavelength range: PDLs can produce laser light in a broad range of wavelengths by simply changing the dye medium, allowing for adaptability to different requirements.
- High output power and peak intensity: Due to the pulsed operation, PDLs generate high peak power, which is useful for many applications that demand intense light sources.
- Short pulse duration: PDLs can produce ultra-short pulses, enabling high precision and minimal collateral damage in applications such as medical treatments and material processing.
- Compatibility with different dye mediums: PDLs can work with a wide range of organic dyes, providing flexibility in terms of wavelength selection and laser properties.
Limitations and Challenges
Despite their advantages, PDLs also face several challenges, including:
- Limited dye stability and chemical degradation: Organic dyes can degrade over time, affecting the performance and efficiency of the laser.
- Need for frequent dye replacement: Due to the limited stability, PDLs often require regular maintenance and replacement of the dye medium.
- High operational costs: The complexity of PDLs and the need for frequent maintenance contribute to higher operational costs compared to other laser types.
- Complexity in designing and maintenance: PDLs require specialized knowledge and expertise for design, operation, and maintenance.
- Limited availability of suitable dyes for specific applications: Not all desired wavelengths can be achieved with currently available dye mediums, limiting their application in certain fields.
Applications of Pulsed Dye Lasers
Pulsed dye lasers have found widespread use in various industries due to their unique properties and adaptability. Some of the most prominent applications are in the medical field, industrial processes, scientific research, and emerging technologies.
Medical Applications
- Dermatology: PDLs have become indispensable in dermatology, thanks to their precision and minimal collateral damage. They are effective in treating a range of skin conditions, including:
- Vascular lesions: PDLs are highly efficient in treating port-wine stains, telangiectasias, and hemangiomas.
- Tattoo removal: The tunable wavelength range allows PDLs to target specific ink colors, breaking down the ink particles for natural removal by the body.
- Scar reduction: PDLs can minimize the appearance of hypertrophic and atrophic scars by stimulating collagen production and remodeling the skin.
- Ophthalmology: PDLs have proven valuable in various eye treatments, such as:
- Retinal photocoagulation: PDLs can precisely target and treat damaged areas of the retina, helping prevent vision loss in patients with diabetic retinopathy or retinal vein occlusions.
- Glaucoma treatment: PDLs can be used for selective laser trabeculoplasty, a procedure that improves fluid drainage and lowers intraocular pressure in glaucoma patients.
Industrial Applications
- Material processing: PDLs offer high precision and control, making them suitable for several material processing techniques:
- Micromachining: PDLs can create intricate patterns and structures on various materials, such as semiconductors, metals, and polymers, enabling the fabrication of microscale devices and components.
- Laser marking and engraving: PDLs can create high-quality, permanent marks on a wide range of materials without causing structural damage.
- Micromachining: PDLs can create intricate patterns and structures on various materials, such as semiconductors, metals, and polymers, enabling the fabrication of microscale devices and components.
- Spectroscopy and chemical analysis: The tunable wavelength range and short pulse duration make PDLs ideal for time-resolved and nonlinear spectroscopy techniques, which provide valuable insights into molecular structures, chemical reactions, and physical processes.
Emerging Applications
- Biophotonics: PDLs are increasingly used in biophotonics research, which involves the use of light to study biological processes, diagnose diseases, and develop advanced therapies.
- Environmental monitoring: The sensitivity and specificity of PDL-based spectroscopy techniques make them promising tools for detecting pollutants and monitoring environmental conditions in real-time.
Scientific Research
- Laser cooling and trapping: Researchers use PDLs to cool and trap atoms and ions, enabling the study of quantum phenomena and the development of ultra-precise clocks and sensors.
- Ultrafast spectroscopy: PDLs’ ultra-short pulse duration allows scientists to investigate ultrafast processes, such as electron dynamics and energy transfer in molecules, on femtosecond timescales.
- Nonlinear optics studies: PDLs enable the exploration of nonlinear optical phenomena, such as harmonic generation and optical parametric processes, which have potential applications in optical communications, imaging, and sensing.
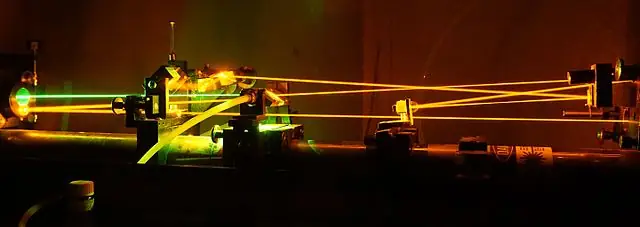
By expanding their applications and overcoming existing limitations, pulsed dye lasers will continue to play a significant role in scientific and technological advancements.
Future Directions and Innovations
Pulsed dye lasers continue to evolve, with ongoing research and development aiming to address existing limitations and expand their applications. Some promising future directions and innovations include:
- Development of new and stable dyes: Researchers are working on creating novel organic dyes with enhanced stability and a broader range of achievable wavelengths, overcoming the current limitations in dye performance.
- Advancements in pumping sources and resonator designs: Improvements in pumping sources and resonator technologies can lead to increased efficiency, reduced operational costs, and enhanced performance of PDLs.
- Integration with other laser technologies: Combining PDLs with other laser systems could enable new capabilities and applications, leveraging the strengths of multiple laser types.
- Potential applications in biophotonics and environmental monitoring: PDLs hold promise for emerging fields such as biophotonics, where they can be used for advanced imaging and diagnostic techniques. Additionally, PDLs could play a crucial role in environmental monitoring, detecting pollutants and other hazardous substances.
Conclusion
Pulsed dye lasers have proven their worth as versatile and powerful tools in a variety of fields. Their unique properties, such as tunable wavelength range, high output power, and short pulse duration, make them an attractive choice for numerous applications. However, the challenges posed by their limitations cannot be ignored. Continued research and advancements in the field are essential to overcome these hurdles and unlock the full potential of pulsed dye lasers. As new innovations emerge, we can expect pulsed dye lasers to play an increasingly prominent role in science, industry, and medicine.