Synchrotron Experiments Illuminate Path to Enhanced Metal 3D Printing
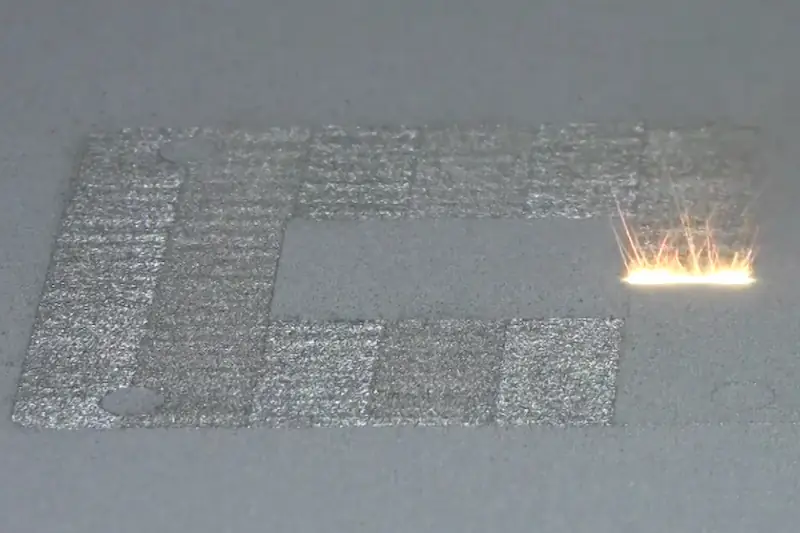
Additive manufacturing, commonly known as 3D printing, has revolutionized the production of various materials, including metals. Despite its transformative potential, metal 3D printing is still in its infancy, with ongoing research focusing on hard metals like steel, softer metals such as aluminum and copper, and essential manufacturing alloys. In an exciting development, scientists from the U.S. National Institute of Standards and Technology (NIST), the KTH Royal Institute of Technology in Sweden, and several other institutions have announced a breakthrough that could grant an unprecedented level of mastery over metal 3D printing.
This collaboration has utilized particle accelerator facilities to investigate the internal structure of steel as it melts and solidifies during the 3D printing process. The findings, published in Acta Materialia, have opened up a valuable computational tool for additive manufacturing professionals, offering them greater predictability and control over the characteristics of printed parts, potentially enhancing the technology’s consistency and feasibility for large-scale manufacturing.
Metal 3D Printing with Particle Accelerator Analysis
In their research, the team used two different particle accelerator facilities to analyze the internal structure of steel as it was melted and then solidified during the 3D printing process. The findings, published in the scientific journal Acta Materialia, are expected to unlock a computational tool for 3D-printing professionals. This tool will offer them a greater ability to predict and control the characteristics of printed parts, making metal 3D printing more reliable and efficient.
Metal 3D printing typically involves welding pools of powdered metal with lasers, layer by layer, into the desired shape. During the initial steps of printing with a metal alloy, the material rapidly heats up and cools off, causing its atoms – which can be a mixture of different elements – to pack into
Understanding the Challenges in Metal 3D Printing
One of the key factors that determine the properties of printed metal parts is the crystal structure formed during the additive manufacturing process. As the material rapidly heats up and cools down, its atoms, which can consist of different elements, pack into ordered, crystalline formations. These crystals directly influence the part’s toughness, corrosion resistance, and other essential properties.
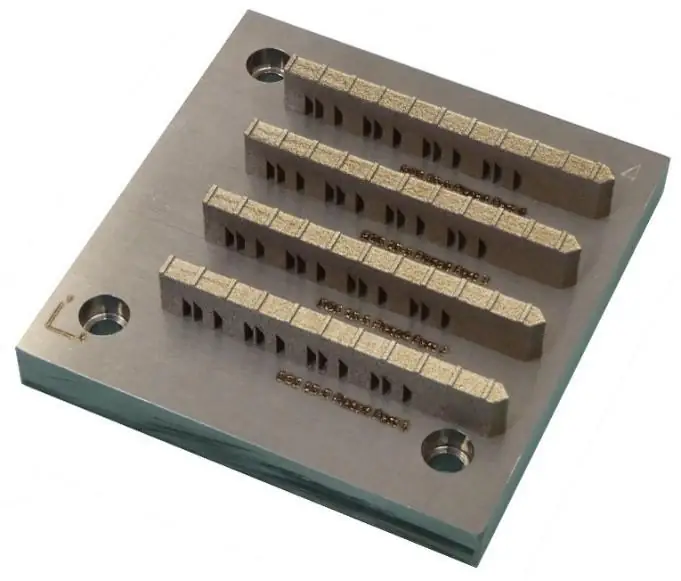
Different crystal structures can emerge, each with their own advantages and disadvantages. NIST physicist Fan Zhang, a study co-author, commented, “If we can control the microstructure during the initial steps of the printing process, then we can obtain the desired crystals and, ultimately, determine the performance of additively-manufactured parts.”
However, researchers have faced challenges in controlling the formation of specific crystal structures during the metal 3D printing process. This lack of control has led to less than desirable results, such as parts with complex shapes cracking prematurely due to their crystal structure. “Among the thousands of alloys that are commonly manufactured, only a handful can be made using additive manufacturing,” Zhang said.
Synchrotron Experiments Shed Light on Crystal Formation
To overcome the challenges of unpredictable solidification during metal 3D printing, the authors of the new study employed powerful X-rays generated by cyclic particle accelerators, called synchrotrons, at Argonne National Laboratory’s Advanced Photon Source and the Paul Scherrer Institute’s Swiss Light Source.
The team’s goal was to learn how the cooling rates of metal, which can be controlled by laser power and movement settings, influence crystal structure. To achieve this, the researchers compared the collected data to the predictions of a widely used computational model developed in the 1980s that describes the solidification of alloys.
“Synchrotron experiments are time-consuming and expensive, so you cannot run them for every condition that you’re interested in. But they are very useful for validating models that you then can use to simulate the interesting conditions,” said study co-author Greta Lindwall, an associate professor of materials science and engineering at KTH Royal Institute of Technology.
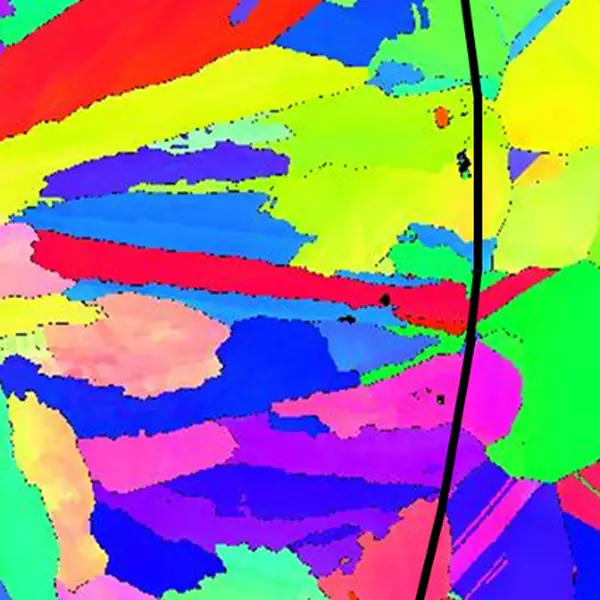
Within the synchrotrons, the authors set up additive manufacturing conditions for hot-work tool steel — a type of metal used to make tools that can withstand high temperatures. As lasers liquefied the metal and different crystals emerged, X-ray beams probed the samples with enough energy and speed to produce images of the fleeting process. The team members required two separate facilities to support the cooling rates they wanted to test, which ranged from temperatures of tens of thousands to more than a million kelvins per second.
The data the researchers collected depicted the push and pull between two kinds of crystal structures, austenite and delta ferrite, the latter being associated with cracking in printed parts. As cooling rates surpassed 1.5 million kelvins (2.7 million degrees Fahrenheit) per second, austenite began to dominate its rival.
Experiment Setup and Findings
To study the process of crystal formation and solidification, the researchers set up additive manufacturing conditions for hot-work tool steel—a type of metal commonly used to create tools capable of withstanding high temperatures. Inside the synchrotrons, lasers were employed to liquify the metal, allowing the team to observe the emergence of different crystal structures.
Thanks to the powerful X-ray beams generated by the synchrotrons, the researchers were able to capture images of the fleeting process with incredible speed and energy. To test a wide range of cooling rates, from tens of thousands to over a million kelvins per second, the team required two separate facilities.
The data collected in the experiments revealed the dynamic interplay between two main types of crystal structures, austenite and delta ferrite. Delta ferrite is known to be associated with cracking in printed parts, which is a significant challenge in metal 3D printing. As the cooling rates surpassed 1.5 million kelvins (2.7 million degrees Fahrenheit) per second, austenite began to dominate its rival, delta ferrite.
Model Validation Leads to Exciting Opportunities in Additive Manufacturing
The experimental findings showed a remarkable agreement with the predictions of the computational model from the 1980s, which has been widely used to describe the solidification of alloys. “The model and the experimental data are nicely in agreement. When we saw the results, we were really excited,” said NIST physicist Fan Zhang.
The validated model now promises to be a reliable tool for materials design not only in traditional manufacturing but also in the additive manufacturing space. This breakthrough has significant implications for scientists and engineers working with metal 3D printing, as it allows them to make more informed decisions about the cooling rates to use during the early stages of the printing process. By selecting the optimal cooling rates, they can promote the formation of the desired crystal structures within their materials, thus making metal 3D printing less unpredictable and more consistent.
Zhang emphasized the importance of this development in accelerating the widespread adoption of additive manufacturing for industrial use. He said, “If we have data, we can use it to validate the models. That’s how you accelerate the widespread adoption of additive manufacturing for industrial use.”
Conclusion
In conclusion, this groundbreaking research paves the way for improved control over metal 3D printing and greater consistency in the resulting printed parts. The successful validation of the computational model offers scientists and engineers a valuable tool to optimize the printing process, making it more feasible for large-scale manufacturing applications. As the technology continues to evolve, we can anticipate a broader range of metal 3D printing applications across various industries, unlocking new possibilities and innovations. Furthermore, this study sets the stage for future research directions, promising further advancements in additive manufacturing and, ultimately, transforming the way we approach the design and production of metal components.