Stokes and Anti-Stokes Emissions: Applications in Spectroscopy, Optoelectronics, and Biomedicine
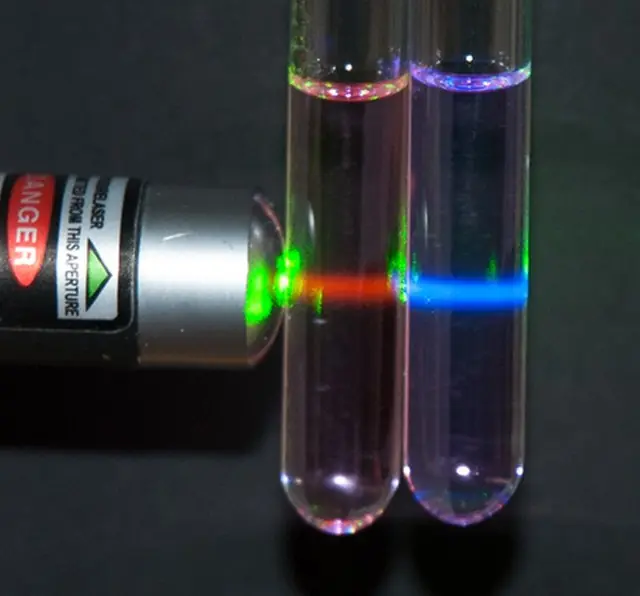
Luminescence, the phenomenon of light emission by a material without heating it, has been the subject of fascination for scientists for centuries. Among the various aspects of luminescence, the concepts of Stokes and Anti-Stokes emissions have captured significant attention due to their applications in a wide range of scientific and technological fields. In this article, we delve into the physical mechanisms that underpin Stokes and Anti-Stokes emissions, and explore their real-world applications.
What is Stokes emission?
Stokes emission is a fundamental process in fluorescence, a phenomenon where a molecule emits light after being excited by absorbing light. In Stokes emission, the material emits photons at lower energy levels than that of the incident photons. This process occurs when a material absorbs a photon of higher energy and then re-emits it after losing some energy through vibrational relaxation or energy transfer processes.
The energy loss through vibrational relaxation arises from the fact that when a molecule is excited, its vibrations become more pronounced. As the molecule relaxes back to its ground state, it releases the excess energy as heat, which is lost to the surrounding environment. Alternatively, the excited molecule may transfer its energy to another nearby molecule, resulting in fluorescence emission from the second molecule.
Stokes emission is used in fluorescence microscopy to visualize specific biological molecules, while in Raman spectroscopy, it helps to identify the chemical composition of a sample. In fluorescence microscopy, a fluorescent molecule is excited by a specific wavelength of light and then emits a lower energy photon, which can be detected by a microscope. In Raman spectroscopy, the frequency of the emitted photon is used to identify the vibrational modes of a molecule, providing information about its chemical composition.
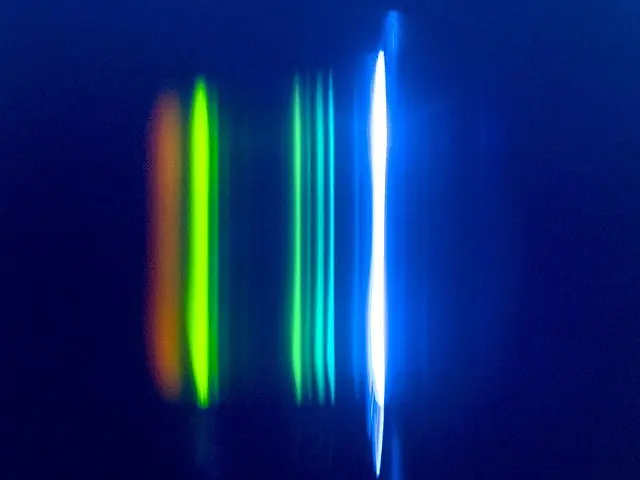
What is Anti-Stokes emission?
Anti-Stokes emission, on the other hand, occurs when a material emits photons at higher energy levels than that of the incident photons. This process can be achieved through phonon-assisted processes or upconversion. In phonon-assisted processes, the excited electrons interact with the phonons (vibrational modes) of the crystal lattice, which results in the emission of a photon with higher energy than the incident photon. In upconversion, two or more rare-earth ions in a crystal are excited, and the energy is transferred to another ion, which emits a photon with higher energy than the incident photon.
Anti-Stokes emission finds applications in various fields, such as improving the efficiency of energy conversion in solar cells and enhancing the sensitivity and resolution of biomedical imaging techniques. In solar cells, upconversion can be used to convert low-energy photons, which are typically not absorbed by the material, into higher-energy photons, which can be absorbed by the material and converted into electricity. In biomedical imaging, upconversion nanoparticles are used to increase the sensitivity and resolution of imaging techniques such as fluorescence microscopy.
Applications of Stokes and Anti-Stokes emissions
The discovery of Stokes and Anti-Stokes emissions has led to numerous breakthroughs in the fields of spectroscopy, optoelectronics, and biomedicine. In optoelectronics, the understanding of these phenomena is crucial for designing efficient light-emitting diodes and lasers. For example, in light-emitting diodes, the emission wavelength is controlled by the energy difference between the conduction and valence bands of the semiconductor, which can be adjusted by incorporating materials that exhibit Stokes or Anti-Stokes emission.
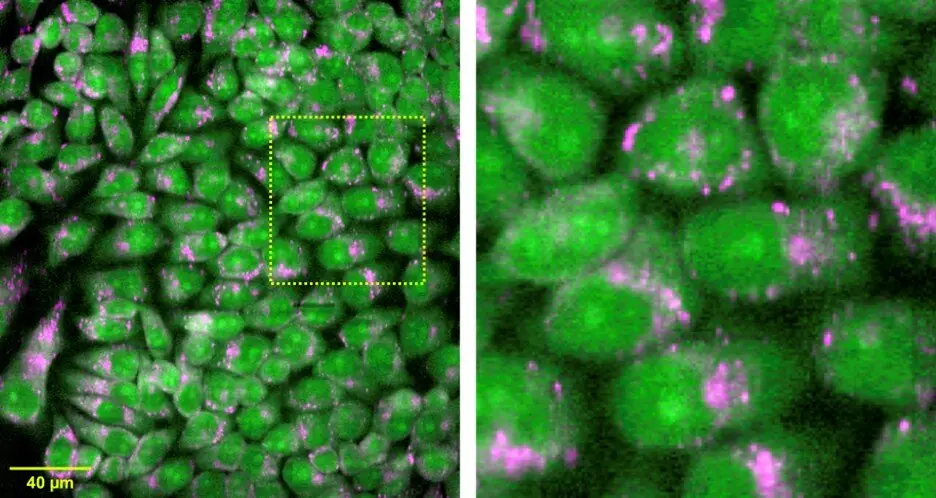
In biomedicine, the fluorescence microscopy technique has revolutionized the field by enabling the visualization of biological molecules and cellular structures with high resolution. For example, in confocal microscopy, a laser is used to excite a fluorescent molecule, and the resulting fluorescence emission is collected by a detector, enabling the visualization of cellular structures with high contrast and resolution. The use of Stokes and Anti-Stokes emission in fluorescence microscopy has further enhanced its capabilities, allowing for the detection of multiple fluorescent molecules simultaneously.
Stokes and Anti-Stokes emissions are also used in Raman spectroscopy, a powerful analytical tool for identifying the chemical composition of a sample. In Raman spectroscopy, a laser is used to excite the sample, and the resulting Raman scattered light is analyzed to determine the vibrational modes of the sample, which provides information about its chemical composition. The use of Stokes emission in Raman spectroscopy can enhance the signal-to-noise ratio and sensitivity of the technique, while Anti-Stokes emission can be used to probe the higher energy vibrational modes of the sample.
In the field of energy conversion, Anti-Stokes emission has found applications in improving the efficiency of solar cells. In conventional solar cells, only a small fraction of the incident sunlight is absorbed and converted into electricity due to the limited absorption range of the material. By using upconversion materials that exhibit Anti-Stokes emission, the low-energy photons that are typically not absorbed by the material can be converted into higher-energy photons that can be absorbed and converted into electricity. This approach has the potential to significantly increase the efficiency of solar cells.
Finally, the use of Anti-Stokes emission in biomedical imaging has also attracted significant attention. Upconversion nanoparticles, which emit light in the visible and near-infrared regions, have been used to enhance the sensitivity and resolution of imaging techniques such as fluorescence microscopy. These nanoparticles can be functionalized with biomolecules such as antibodies, enabling the targeted imaging of specific cellular structures or biomolecules. Furthermore, the use of upconversion nanoparticles can reduce the autofluorescence background, enabling the detection of weak fluorescent signals.