Laser-induced breakdown spectroscopy (LIBS) for material analysis
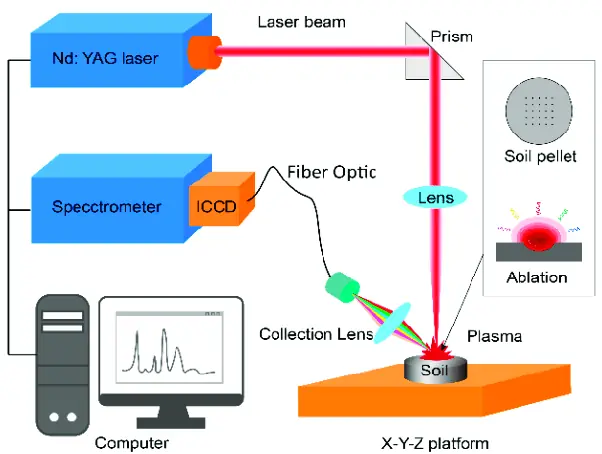
Laser-induced breakdown spectroscopy (LIBS) is a powerful analytical technique that has gained popularity in recent years for its ability to provide rapid and non-destructive analysis of a wide range of materials. LIBS has been utilized for material identification, elemental analysis, and trace element detection, and has proven to be a valuable tool in a variety of applications. In this article, we will explore the theoretical background, advantages, limitations, and future directions of LIBS in material analysis.
The basic principles of LIBS involve focusing a high-energy laser beam onto a sample, causing a plasma to form. The plasma emits light, which is then collected and analyzed using a spectrometer. This spectral analysis provides information about the elemental composition of the sample. LIBS instrumentation typically consists of a laser, a spectrometer, and a data acquisition system. LIBS spectral analysis involves identifying peaks in the spectrum and matching them to known elemental lines.
LIBS has a wide range of applications in material analysis, including material identification, elemental analysis, and trace element detection. Material identification can be performed by comparing the spectrum of an unknown material to a database of known spectra. Elemental analysis involves quantifying the concentration of elements in a sample, while trace element detection can detect elements present at very low concentrations.
One of the key advantages of LIBS is its non-destructive nature, which allows for analysis of delicate samples without damaging them. Additionally, LIBS provides high sensitivity and specificity, allowing for detection of even trace amounts of elements. LIBS is also a rapid analysis technique, with results typically obtained in seconds to minutes.
However, LIBS is not without limitations and challenges. One major limitation is the effect of sample matrix on spectral analysis, which can result in inaccurate quantification of elements. Calibration and sample preparation issues can also impact accuracy. Data analysis and interpretation can be challenging, especially when dealing with complex spectra.
Despite these challenges, LIBS has been successfully applied in a variety of fields. For example, in archaeology, LIBS has been used to identify pigments in ancient artifacts, while in environmental science, it has been used to detect trace elements in soil samples. LIBS has also been compared to other analytical techniques, such as X-ray fluorescence (XRF) and inductively coupled plasma mass spectrometry (ICP-MS), with promising results.
Looking to the future, current research and development in LIBS is focused on improving accuracy and expanding applications to new fields. Potential applications include biomedical research, where LIBS could be used to analyze tissues and fluids, and materials science, where it could be used to analyze the composition of alloys and polymers. Technological advancements, such as miniaturization of instrumentation and improved data analysis algorithms, are also expected to increase the versatility and usefulness of LIBS.
In conclusion, LIBS is a powerful analytical technique that has proven to be a valuable tool in material analysis. With its non-destructive nature, high sensitivity and specificity, and rapid analysis capabilities, LIBS has a wide range of applications and is expected to continue to grow in importance in the future.